This article focuses on the comparative analysis and synergistic potential of air-based and biogenic CO2 capture techniques, highlighting their central roles in advancing carbon management practices.
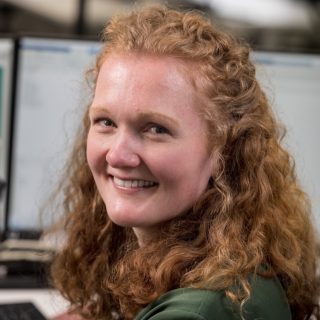
A comparative look at Direct Air Capture and CO2 capture from biogas upgrading
Jun 25 2024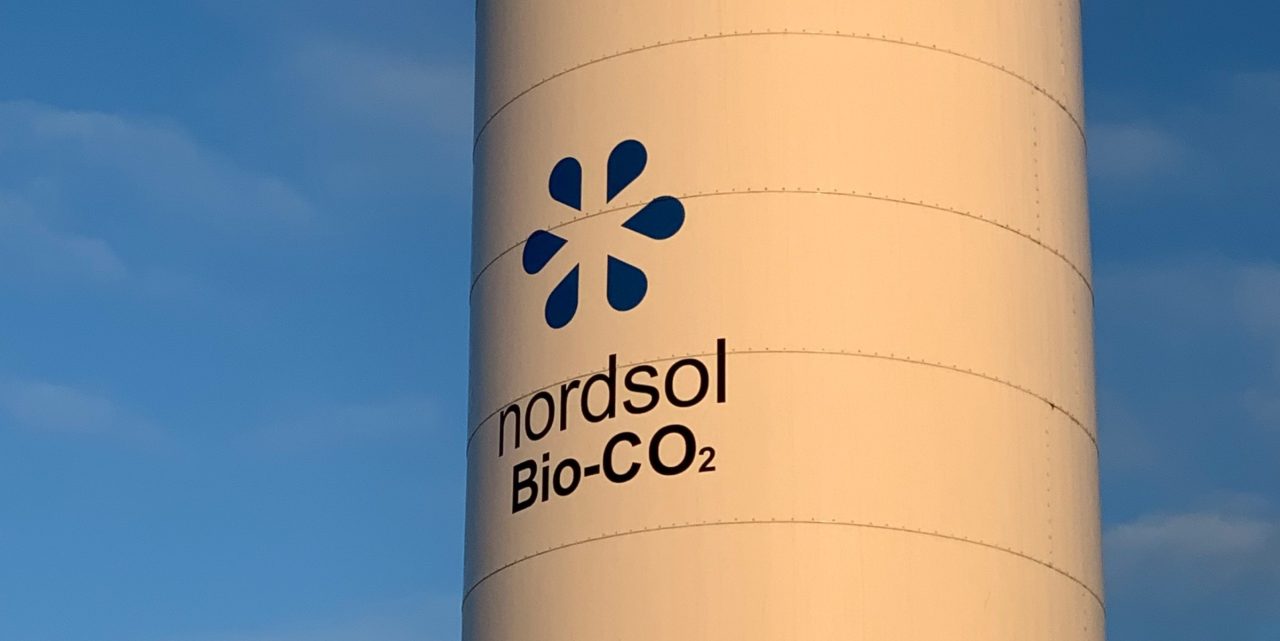
In our collective effort to address climate change, carbon capture technologies have become pivotal in achieving both European and global climate objectives. Direct Air Capture (DAC), which involves capturing carbon dioxide directly from the atmosphere, is a relatively recent innovation that has attracted a lot of attention due to its potential to significantly reduce atmospheric CO2 levels. A less well-known but equally important method involves capturing CO2 during biogas upgrading, where CO2 is separated from biomethane – a renewable energy source derived from organic materials. The biogenic CO2 generated in this way, being free from climate-relevant emissions, presents a valuable resource for various applications.
The DAC sector is rapidly expanding as new companies explore various technologies. Similarly, interest in biogenic CO2 capture is on the rise. At Nordsol, we acknowledge the significance of this approach and are actively contributing to this growing field.
Direct air capture technology represents a considerable advancement in our efforts to reduce atmospheric CO2 levels. This innovative method involves extracting CO2 directly from the atmosphere, where it exists at a concentration of about 0.04% or 410 ppm. One of the key strengths of DAC is its flexibility in terms of location – it can be set up in a variety of environments due to its minimal land and resource requirements. This characteristic positions DAC as a potentially global solution for atmospheric CO2 reduction.
There are currently two main methods of capturing CO2 from the atmosphere: chemical absorption, where chemical solvents such as amines absorb CO2, which is then released by heating, and temperature-pressure swing adsorption (TPSA), where CO2 is adsorbed onto a solid material and later released through temperature and pressure adjustments. Each method has its own challenges, particularly in terms of the energy required for effective operation [1].
The energy-intensive nature of DAC becomes evident when considering the minimum theoretical energy required for separating CO2 from gas streams of varying concentrations. This relationship is illustrated in Figure 1, which shows the higher energy requirement for capturing CO2 from lower concentration sources – a fundamental challenge for DAC technology.
Figure 1: This graph compares the minimal theoretical energy required to separate CO2 from gas streams (represented by the purple line, indicating Gibbs free energy) against the actual energy requirements for various CO2 capture methods (green line). It includes data for Direct Air Capture (DAC), natural gas combined cycle (NGCC) power plants, advanced supercritical (ASC) coal power plants, and carbon capture and storage (CCS) from cement production, adapted from [4].
Currently, the cost of DAC technology is between 600 and 1000 euros per ton of CO2 captured [1]. This figure relates solely to the capture process and does not include the additional costs of liquefying and transporting the captured CO2. According to forecasts, advances in DAC technology could reduce these costs to less than 150 euros per ton by 2050 [2]. In addition, the technology requires significant sustainable power, necessitating a large land area for energy sources, such as solar or wind farms, to operate efficiently and sustainably.
One of the key strengths of DAC is its flexibility in terms of location – it can be set up in a variety of environments due to its minimal land and resource requirements.
As of the end of 2023, the total DAC capacity in the European Union stood at 0.51 Mt CO2/year. Projections for 2026 indicate an increase in capacity to between 1.1 and 2.1 Mt CO2/year, including contributions non-EU member Norway. Looking further ahead, the European Commission has set an ambitious target of capturing 5 Mt CO2 per year by 2030 [2].
This emerging technology, while still developing, holds considerable promise for future carbon reduction strategies, offering a solution that can be tailored to various geographic and environmental conditions.
Capturing CO2 from biogas installations represents a significant opportunity in carbon management and stands out for its practicality and effectiveness. The technique focuses on CO2 produced during the anaerobic digestion of organic materials, leading to the creation of biomethane—a renewable substitute for fossil methane—and a stream of biogenic CO2. The latter, free from climate-relevant emissions, emerges as a crucial resource for a range of applications, marking an essential step toward sustainable carbon management.
Beyond biogas upgrading, capturing CO2 from the combustion or gasification of biomass in bioenergy production is one of several additional pathways that contribute to making biogenic CO2 usable for sustainable development.
A key aspect of biogenic CO2 capture is the sustainability of biogas production, which is influenced by the choice of biomass used. In recent years, there has been a shift towards more environmentally sustainable feedstocks, such as agricultural residues, municipal biowaste, and sewage sludge, away from reliance on food and feed crops [3]. This shift is not only in line with the sustainability criteria of the Renewable Energy Directive, but also supports the reduction of greenhouse gas emissions and the wider goals of sustainable climate change mitigation. The use of waste biomass and agricultural residues reduces environmental and social impacts and steers bioenergy production in a more sustainable direction.
One advantage of CO2 capture from biogas is the high concentration of CO2 in the gas, typically ranging from 30-50% (Figure 1). This concentration enables efficient capture processes. Capturing biogenic CO2 can be considered cost-neutral as it is a by-product of biomethane or bio-LNG production, with its purity largely depending on the biogas upgrading technology employed. The primary methods—adsorption, chemical absorption, membrane separation, and cryogenic separation—are all capable of producing high-purity CO2. Traditional methods, such as using liquid solvents, require regeneration at high temperatures, additional equipment, and chemicals, potentially leading to waste streams.
One advantage of CO2 capture from biogas is the high concentration of CO2 in the gas, which enables efficient capture processes.
Furthermore, the cyclic nature of adsorption can result in fluctuations in gas flow and temperature, impacting system stability. Membrane separation is notable for its continuous, energy-efficient operation that requires neither heat nor chemicals and produces no waste, thereby simplifying CO2 capture. Although cryogenic separation directly liquefies CO2, it is more energy-intensive compared to other methods. For technologies other than cryogenic separation, integrating a liquefaction unit incurs additional costs ranging from 50 to 150 euros per ton for liquefying captured CO2. While all methods can produce high-quality CO2 suitable for liquefaction, intelligently integrating these processes is essential to ensure operational ease, minimize CO2 losses, and reduce energy consumption.
In 2020, the biogas and biomethane production in Europe indicated a theoretical potential of capturing 24 Mton of biogenic CO2, with approximately 3.9 MtCO2/year already being separated during the biogas upgrading process [3]. As production is expected to double by 2030, the potential for CO2 capture could increase to 46 Mton. Assuming these projections hold true, this would exceed DAC capacity by more than nine times. Looking ahead to 2050, this potential is projected to reach 124 Mton, equivalent to 3% of the EU-27’s greenhouse gas emissions in 2020 [3].
Such projections underscore the vital role of biogenic CO2 capture in the overarching strategy for achieving climate neutrality, offering a cost-effective, sustainable, and immediately actionable solution within the broader spectrum of carbon management technologies.
Membrane separation is notable for its continuous, energy-efficient operation that requires neither heat nor chemicals and produces no waste, thereby simplifying CO2 capture.
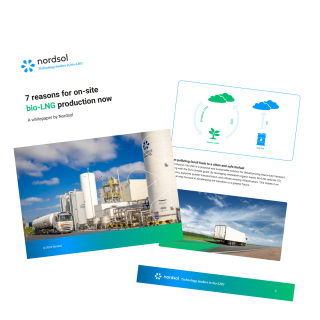
Learn how biogas producers significantly enhance both the ecological and economic value of their biogas through bio-LNG production, utilizing Nordsol’s innovative biogas liquefaction technology.
The fight against climate change requires a variety of solutions, with both Direct Air Capture (DAC) and CO2 capture from biogas upgrading plants playing crucial roles. DAC, often highlighted in literature and media, is recognized for its broad potential. CO2 capture from biogas plants, though receiving less public attention, has shown great promise and provides an immediate and efficient strategy. These technologies complement each other, each playing an important role in the common global strategy to combat climate change.
At Nordsol, we developed a technology that smoothly integrates CO2 capture into bio-LNG production, and our technology highlights the economic and environmental synergies achieved through this process. Our membrane concept for treating biogas combines very well with the liquefaction of biomethane and biogenic CO2, resulting in no losses of CO2 or CH4 during production. Although bio-LNG production remains our primary focus, the capture and liquefaction of CO2 not only helps to improve the environmental impact of our operations but also increases the profitability and attractiveness of our technology. By intelligently combining CO2 liquefaction and bio-LNG production, we can streamline the process, minimize losses, and improve overall efficiency. This approach embodies the principles of a circular economy that optimizes the use of resources while minimizing environmental impact.
By intelligently combining CO2 liquefaction and bio-LNG production, we can streamline the process, minimize losses, and improve overall efficiency.
Our Amsterdam demonstration plant already captures approximately 5500 tons of CO2 annually, equivalent to the emissions from around 1300 average cars per year. As we expand our projects, we expect this contribution to CO2 reduction to increase significantly in the future. While our current efforts are just the beginning in the broader context of climate action, they are crucial and impactful steps toward realizing the full potential of available technologies. By combining the possibilities of CO2 capture from biogas with bio-LNG production, we are confident that together, we will achieve the EU’s carbon neutrality goal.
[1] Sabatino, F., Grimm, A., Gallucci, F., van Sint Annaland, M., Kramer, G. J., & Gazzani, M. (2021). A comparative energy and costs assessment and optimization for direct air capture technologies. Joule, 5(8), 2047-2076.
[2] McQuillen, J. (2022). European CO2 availability from point-sources and direct air capture. Report for: Transport & Environment. 2022
[3] EBA 2022. EBA Statistical Report 2022. Brussels, Belgium, November 2022.
[4] Grimm, A., Kramer, G. J., Gazzani, M., Sabatino, F., van Sint Annaland, M., Gallucci, F. (2019). Process modeling and optimization for production of carbon-neutral solar fuels. Poster presented at GHGT-13. Switzerland.
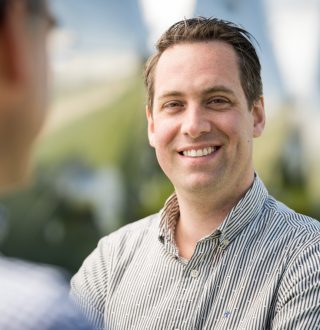
your next big step?
From technical expertise to operational support, we guide you every step.
Let's explore together. Take our quick scan or contact Rob now.